Introduction
Techniques for mutation detection in disease-related genes need to be sensitive and specific. Therefore the ideal method to use for mutation analysis particularly if large numbers of DNA fragments need to be analyzed should be sensitive, non-hazardous, relatively inexpensive, and semi- or fully automated to minimize labor and laboratory costs. Denaturing high-performance liquid chromatography (DHPLC) had been shown to meet these criteria for a growing number of applications in disease-related genes, such as CFTR (1), TSC1 (2), hereditary non-poliposis colon cancer (3), mutations in haemochromatosis (4), factor IX and neurofibromatosis type 1 genes (5), BRCA1 and BRCA2 (6, 7).
This technology, which is based on heteroduplex detection, allows for automated identification of single nucleotide polymorphism (SNP) and small deletions or insertions. Heteroduplex profiles are easily distinguished from homoduplex peaks (8, 9) and thereby provide a reliable means for mutation scanning and discovery. DHPLC has been shown to clearly resolve mutations in various genes with detection rates ranging from 92.5% to 100% (10, 11). Furthermore, it is reported in the literature that the sensitivity of detection by DHPLC is higher than for alternative gel-based analysis techniques; that is, mutations not detected by gel-based techniques could be detected by DHPLC (12, 13).
There is no previous report available which addresses the feasibility of mutation or polymorphism detection by DHPLC analysis for estrogen research β gene (ESR2) gene in infertile male patients. We therefore decided to evaluate the sensitivity and specificity of DHPLC for sequence analysis of the ESR2 gene in these patients. The physiological and pathophysiological roles of estrogens in men have gained increasing attention and are now known to have profound effects on not only the female, but also the male reproductive system. In men, estrogens are synthesized from testosterone mainly in testis, through the action of aromatase cytochrome p450 (14). Estrogen signaling in the cell is mediated by estrogen receptors, of which at least two subtype exist, ESR1 and ESR2. Estrogens, as steroid hormones, act through specific nuclear estrogen receptors (ER), which bind in dimeric form to specific sequences of DNA, in the regulatory region of target genes, and recruit coactivators and corepressors, leading to changes in the rate of transcription of estrogen-regulated genes. These coregulators bind mostly to the ligand-binding domain (15-17). These receptors are present in multiple organ systems, and are important in human development. Two isoforms of the human ER,
ESR1 and
ESR2, occur, each with distinct tissue and cell patterns of expression, modest overall sequence identity, and with different affinities for different response elements and can therefore yield different transcriptional effects at the same site (18-22).
The recently discovered ESR2 is expressed in both Leydig cells and Sertoli cells in the human testis (23), as well as in most germ cells from spermatogonia to elongating or elongated spermatids. The role of classical ESR1 has been thoroughly studied but there are not many reports of ESR2. Recently, several sequence variants of the ESR2 gene have been described (24). In this study we tried to evaluate the DHPLC analysis for detection of new sequence variations and their relation with male infertility.
Materials and Methods
Patients
DNA samples were obtained from 96 unrelated infertile men referred to Uremia' University Hospitals presenting with sperm concentrations below 5x10
6 /ml in at least two ejaculates.
As normal control, an unselected group of 96 fertile men, without genital abnormalities and at least one healthy child were chosen. Informed consent was obtained from all subjects or their parents, according to protocol of ethical review board of Uremia University.
PCR amplification
Genomic DNA was extracted from EDTA-preserved blood samples and isolated according to standard procedures (8). Samples used for mutation screening were amplified in 50ml reaction volumes containing approximately 200ng DNA , MgCl
2 (25mM) 3ml, dNTP mixture (1.25Mm) 4ml, AmpliTaq GoldTM (5U/ml) 0.5ml, and 50pmol of each primer. In ESR2 exon1, 2 and 3 the amount of MgCl2 (25mM), and dNTP mixture were increased to 4ml and 6ml respectively. Cycle conditions used for amplification were 15 min at 95°C for denaturation, 35 cycles of 30 second at 95°C, 30 second at 56°C and 55 second at 72°C. All amplifications were finished by a prolonged extension step of 4 min at 72°C.
Primers were chosen so that an average length of fragments was between 250 and 600 bp (table I).
DHPLC analysis was carried out on an automated HPLC device equipped with a DNA separation column (WAVE: Transgenomic, San Jose, CA, USA) according to the manufacturer’s protocol. Chromatography was performed with buffers A, aqueous solution of 0.1M triethylammonium acetate (TEAA), and B, 0.1M TEAA in 25% acetonitrile (ACN). ACN (Transgenomic), TEAA (Transgenomic), and water used for buffer preparation were of HPLC grade. Eluent conditions for analysis were determined using the Wavemaker™ software (Transgenomic). The eluent flow rate for all analyses was 0.9ml/min. Chromatograms were recorded at a wavelength of 260nm. Analyses under nondenaturing conditions were conducted at 50°C. Mutation detection under partially denaturing conditions was performed at the temperature indicated for each chromatogram.
10ml of each PCR product (containing 10–100ng DNA) was denatured at 95°C for 5 min and then gradually reannealed by decreasing the sample temperature from 95 to 65°C over a period of 30 min. Analysis took in general approximately 7–8 min including column regeneration and re-equilibration to starting conditions. The column mobile phase consisted of a mixture of 0.1M triethylamine acetate (pH 7.0) with (buffer B) or without (buffer A) 25% acetonitrile.
Optimal column temperatures were calculated based upon the melting temperature of the PCR product in either the Transgenomic Wavemaker™ software or in the Stanford melt program (http://www.insertion.stanford.edu/melt.html), as a reference.
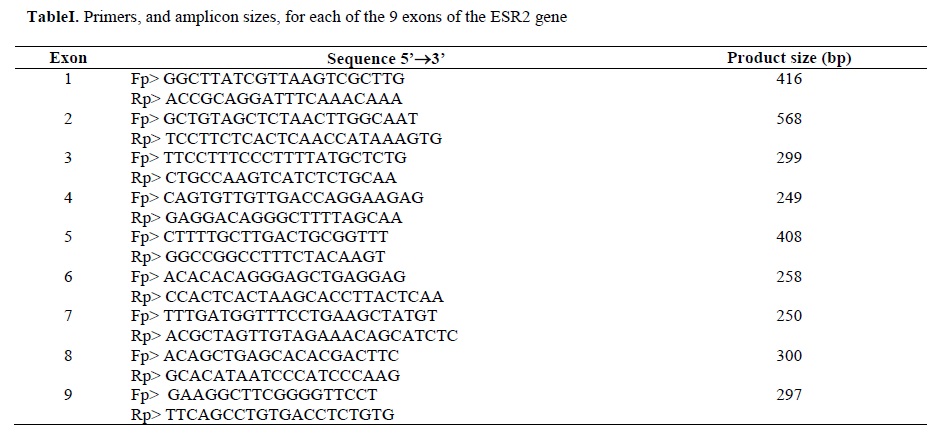
Fragments showing an abnormal DHPLC pattern were investigated for identification of sequence variants by automated sequence analysis on the ABI Prism 310. This was performed according to the manufacturer’s protocol using a reamplified PCR product of the abnormal fragment (forward and reverse). The sequence variants were classified according to international databases.
PCR amplicons were purified using the QIAquick PCR Purification Kit (Qiagen, Valencia, Ca.). DNA concentration was determined by spectrophotometry. Cycle sequencing extension products were created in a final volume 20 ml reaction using 12.5ml H
2O, 0.5ml forward or reverse primer at 10mg/ml, 0.5ml template DNA, 4ml Big Dye Terminator Ready Reaction mix V1.1 (PE-ABI, Foster City, Ca.) and 2ml 5X Big dye buffer. Cycle sequencing conditions consisted of an initial denaturation step at 96°C for 1 min. followed by 25 cycles of 96°C for 10 s, 50°C for 5 s, and 60°C for 4 min. Unincorporated dye and other contaminants were removed with ethanol precipitation procedure. Data was analyzed with the Sequencers software (Genecodes Inc. Ann Arbor, Michigan).
Statistical analysis
The distributions of ER polymorphisms were compared between the patient group and controls using Fisher's exact test. All statistical tests were two sided. p<0.05 was considered statistically significant.
Results
DHPLC evaluation of 96 infertile patients' (8 with testis degeneration) ESR2 gene, revealed one sequence variations in five patients. They all had double peaks or an atypical fragment configuration indicating heteroduplex formation. For patients with double peaks or even tetra peaks in DHPLC evaluation, sequence variations could be detected. All the five patients showed heterozygous mutation in intron 8 near intron splicing site (IVS 8–4G<A) (Figure1). The matched control population of 96 individuals presented only the normal variant. The difference between the 2 groups was statistical significant (p=0.021).
Discussion
Mutation detection by DHPLC, as it is presented in this context, is a high-throughput, time saving, and economical tool for mutation screening. DHPLC provides information about whether a mutation is present. In order to determine the specific nature of the mutation, however, PCR products in question need to be sequenced.
Up to 96 samples can be analyzed in an automated fashion in a single series of runs. About 200 individual samples can be screened for the presence of mutations per day using DHPLC. By applying this method five patients showed heterozygous mutation in intron 8 near intron splicing site (IVS 8–4G<A).
This sequence variation or polymorphism has previously been associated with several conditions, such as bone mineral density in postmenopausal women (25-28), endometrial cancer (29) and systemic blood pressure (30). In addition, estrogens can influence serum androgen levels. This has been recognized in women taking oral contraceptives, or receiving estrogen replacement therapy during menopause, resulting in reduced serum levels of ovarian and adrenal androgens (31-33). Consequently, polymorphisms of the ESR1 and ESR2 were studied in a population-based cohort of 270 women, where long (CA)
n polymorphisms have been associated to lower levels of testosterone (15).
Since this variation was not present in the control population with 192 chromosomes in the present study, but it was detected in five of our patients (frequency 5/192=2.6%), therefore it is possible to conclude that there has a statistic significant association between this polymorphism and male infertility in our study (p<0.05).
Yet unable to present any functional explanation for this variation, it is possible that this sequence variation is in linkage disequilibrium with other regulatory sequence variations that may affect gene expression or function, ultimately leading to infertility; or they can cause different structural folds of mRNA and or altered splice, which may possess biological implications, leading to a higher susceptibility to infertility.
Since the polymorphism is located near intron-exon splicing site it could affect the splicing procedure.
Conclusion
In conclusion, the importance of the sequence variation that we’ve found can not yet be understood nor underestimated. Screening the cDNA or, in the case of suspected splice mutations, using accurate models of splice strength, would be needed to predict the consequences of these genetic alterations.
Acknowledgments
We thank the families for their cooperation in the study and also we would like to thank Dr. Ebrahimpour Azar for kindly providing some of the material in this project. This study was funded in part by the Uremia's Medical Science University research deputy.